The Biohybrid Solar Power Plant (BISON)
Evolution took billions of years to optimize photosynthesis of cyanobacteria and plants, forming the ultimate basis of all life on earth. The two key steps of photosynthesis are the conversion of light energy into chemical energy via water-splitting and the use of this chemical energy to fix atmospheric carbon into biomass. While carbon fixation is slow and inefficient, photosynthetic water-splitting is a highly efficient and fast process. In contrast to traditional (photo-)biotechnology, which aims at harvesting products downstream of carbon fixation, BISON aims at using bio-photovoltaics (BPV) to synthesize products directly from the water-splitting and upstream of all limiting steps of metabolism.
BPV utilizes cyanobacterial photosynthesis to split water using sunlight and releasing electrons. These electrons can transfer directly to an electrode and initiate valuable chemical reactions, such as hydrogen production or carbon dioxide (CO₂) reduction. BPV has several unique advantages over conventional electrolytic systems and conventional photo- biotechnological approaches:
- The system does not need high-purity water or costly catalysts
- It operates at low voltages, reducing energy consumption.
- CO₂ can be converted into value-added products through biocatalytic or electrochemical processes, fostering a circular economy.
- The system functions with only sunlight, water, CO₂, and minimal nutrients, enabling resource conservation.
By advancing BPV technology, BISON contributes to sustainable energy solutions, potentially transforming industrial CO₂ usage, reducing energy input needs, and establishing renewable pathways for hydrogen and chemical production. Unlike previous projects, BISON does not want to stop at the lab scale and take the next step of testing a BPV system under long-term, outdoor applications.
The Impact
BISON directly contributes to the shift from a coal-based economy to a circular economy by pioneering sustainable technologies that integrate renewable energy and CO₂ utilization. Using sunlight, the project develops hydrogen production and CO₂ conversion into valuable products like formic acid, laying the foundation for circular value chains. These innovations align with regional transformation priorities, such as diversifying the economy and fostering new industries. BISON’s technology can be implemented by small and medium-sized enterprises (SME) and hence supports local creation of value, creating skilled jobs and fostering international collaboration. It also promotes climate neutrality by reducing CO₂ emissions and eliminating the need for resource-intensive catalysts, advancing sustainable resource management.
The Herd
This ambitious initiative brings together an interdisciplinary team of experts from material sciences, microbiology, biochemistry, and engineering from UFZ and University of Leipzig. Together, they aim to scale the BPV system from laboratory setups to real-world applications, advancing energy efficiency, environmental sustainability, and resource management.
For more information on the heads behind the science please refer to the individual work packages (WP).
WP1 focuses on developing and optimizing materials for electrodes and membranes to maximize the energy efficiency of the BPV system. Innovative surface modifications, such as electron-beam techniques, are used to enhance material functionality and durability. These materials undergo rigorous testing for performance and scalability, ensuring they can meet the demands of real-world applications. Collaborating with other work packages, WP1 integrates novel mediators and biofilm technologies to refine subsystem designs. This foundational work contributes to the efficiency and reliability of the entire BPV system, establishing a benchmark for future biohybrid energy technologies. Work on WP1 is conducted in the group of Physical Chemistry of Complex Systems and Technology at the University Leipzig.

Dr. Ales Charvat
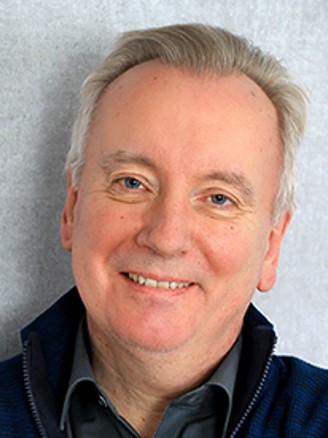
Prof. Bernd Abel
WP2 develops advanced redox mediators to improve electron transfer between photosynthetic microorganisms and electrodes. These mediators are synthesized to optimize solubility, mobility, and compatibility with biological systems. By targeting specific electron transport proteins, the mediators enhance reaction efficiency while maintaining biological stability. Advanced imaging techniques, such as fluorescence microscopy, are employed to analyze mediator interactions in real-time. WP2 also explores covalent binding of mediators to electrode surfaces, ensuring precise and efficient electron flow. This work is critical for improving the overall performance and scalability of the BPV system. Work on WP1 is conducted in the group for Coordination Chemistry at the University Leipzig.
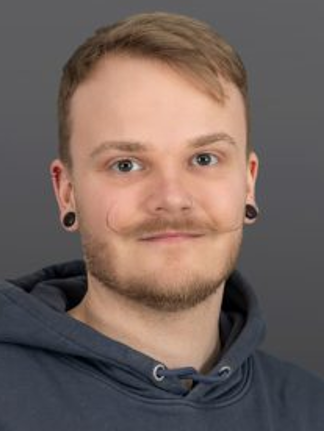
M.Sc. Lennart Günzel
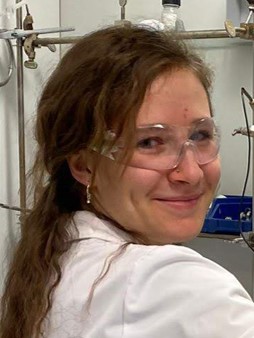
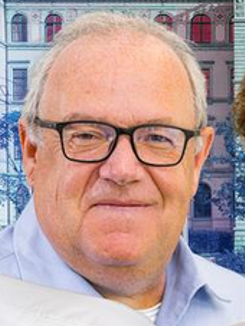
Prof. Berthold Kersting
WP3 investigates the complex interactions between microorganisms, mediators, and electrodes to optimize the BPV system’s biological and electrochemical components. Using system biology approaches, researchers analyze cellular responses to different reaction conditions, focusing on productivity and stability. Data from metabolic and photosynthetic studies help identify the best combinations of organisms, mediators, and materials. The results inform the design of robust and efficient BPV systems, enabling seamless integration across work packages. This work ensures that the biological components remain highly productive and resilient, even under challenging conditions. WP3 is conducted in the group of Systems Biotechnology at UFZ.
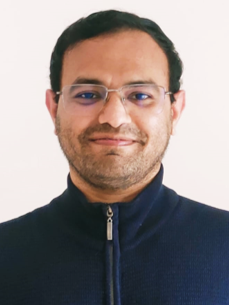
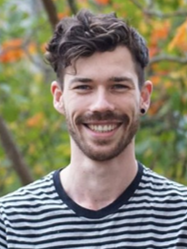
M.Sc. Hans Schneider
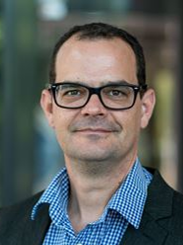
Prof. Jens Krömer
WP4 focuses on designing modular µBPV systems for detailed analysis of reaction dynamics and photosynthetic efficiency. These chip-sized reactors offer a controlled environment for testing material properties, mediator performance, and cellular responses. Utilizing advanced 3D-printing techniques, the miniaturized systems allow rapid prototyping and real-time observation of reaction processes. The insights gained from these experiments support the refinement of larger BPV systems. WP4 bridges the gap between theoretical models and practical applications, providing a scalable platform for optimizing biohybrid energy systems in collaboration with other work packages. WP4 is conducted in the departmant of Solar Materials Biotechnology at UFZ.
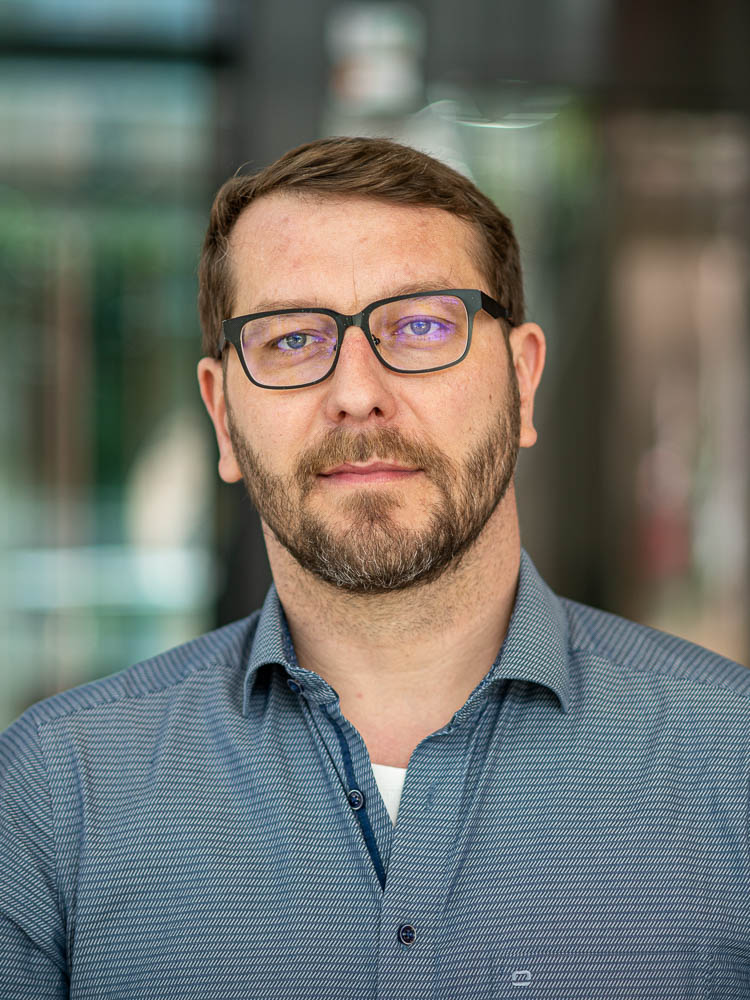
Dr. Martin Herzberg
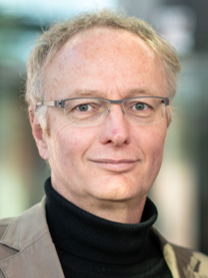
WP5 scales insights from µBPV systems to laboratory reactors, enabling comprehensive testing of materials, mediators, and microorganisms. The package evaluates various combinations to identify optimal setups for high performance and reproducibility. By refining process kinetics and electrochemical parameters, WP5 ensures that the BPV system operates efficiently under lab conditions. This work also generates consistent samples for system biology analyses in WP3, ensuring integration and data-driven improvements. The outcomes of WP5 lay the groundwork for scaling the BPV system to field applications, bridging laboratory innovations with practical deployment. WP5 is conducted in the group of Biophotovoltaics at UFZ.
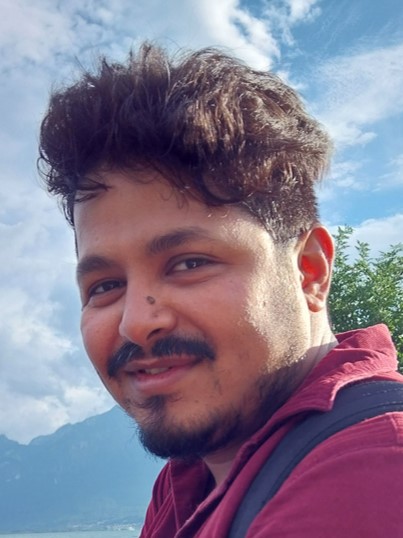
Dr. Abdul Quadir
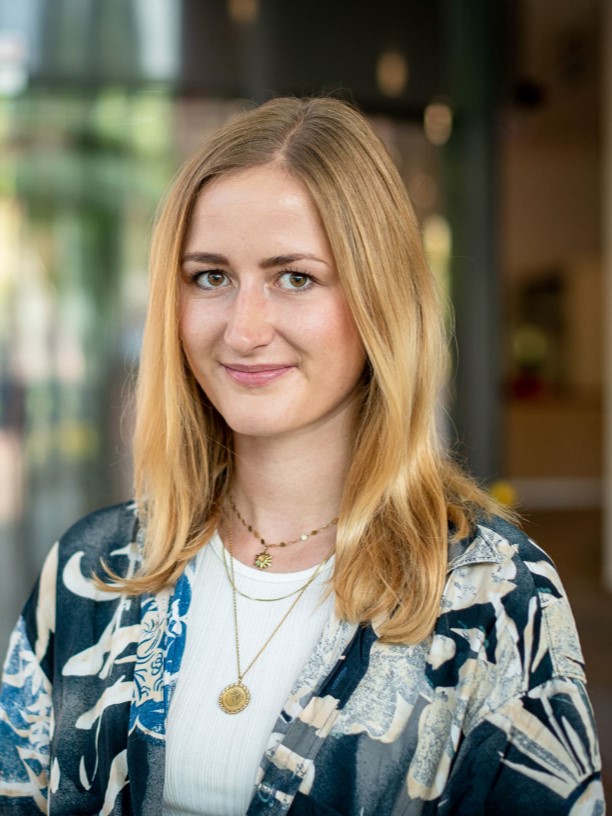
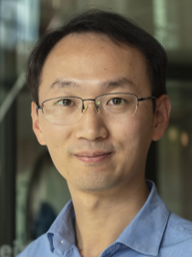
Dr. Bin Lai
WP6 aims to stabilize the biological catalysts by developing robust biofilm structures that optimize light capture and productivity. Researchers investigate growth parameters, biofilm properties, and their interaction with electrode materials to enhance stability and efficiency. The work includes testing naturally occurring microorganisms and adapting biofilms for both laboratory and outdoor environments. Collaboration with other work packages ensures that materials and mediators are compatible with the biofilm system. WP6 contributes to long-term system resilience and scalability by maximizing light utilization and maintaining catalyst performance. WP5 is conducted in the group of Catalytic Biofilms at UFZ.
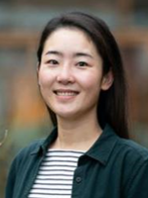
Dr. Yiru Wang
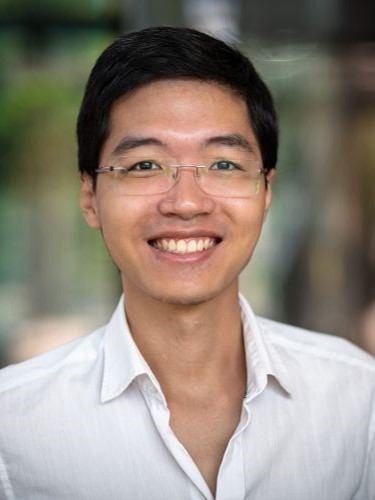
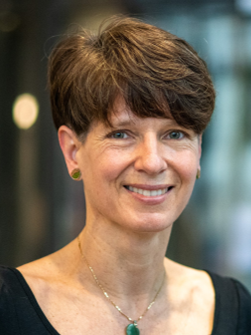
Prof. Katja Bühler
WP7 designs and tests electrochemical modules that integrate seamlessly with the BPV system. These modules facilitate efficient energy conversion processes, focusing on optimizing anode and cathode reactions. Utilizing advanced prototyping techniques, researchers develop cost-effective and scalable solutions. The work emphasizes modularity, allowing for easy expansion and integration into larger systems. WP7 also investigates material performance and operational parameters, ensuring the modules meet real-world requirements. This work is essential for achieving the project’s goals of scalability and practical deployment in diverse environments. WP3 is conducted in the group of Electrobiotechnology at UFZ.
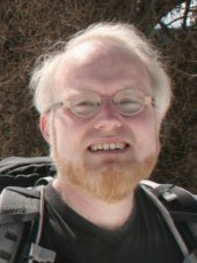
Dr. Matthias Schmidt
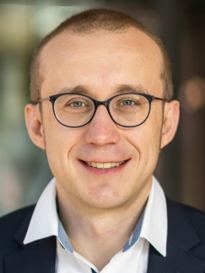
WP8 integrates individual modules into a cohesive system, focusing on energy efficiency and adaptability to varying light conditions. Researchers identify key operational parameters to optimize system performance, including energy and electron transfer efficiencies. By testing setups with up to one square meter of active surface area, WP8 evaluates scalability and robustness. The package also incorporates storage and processing solutions for electrochemical products, ensuring seamless operation under real-world conditions. This work package bridges laboratory advancements and field-ready systems, enabling the transition to practical energy applications. WP8 is conducted in the group for Thermodynamics of electroactive microorganisms at UFZ.
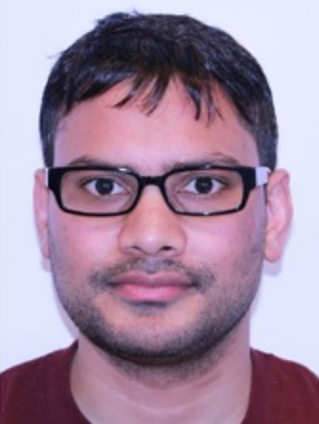
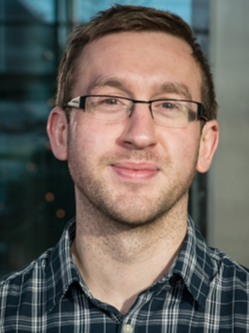
The results of all WPs merge in WP9, which realizes and validates the BPV system in outdoor settings, ensuring scalability and operational durability. This package coordinates contributions from all work packages to build and deploy a pilot system. Researchers test the system’s performance under real-world conditions, refining process parameters and assessing long-term reliability. By scaling up and validating the system, WP9 prepares the technology for commercial applications. This package represents the culmination of the BISON project, translating cutting-edge research into a sustainable and impactful energy solution. WP9 is under lead management of the group of Systems Biotechnology at UFZ.
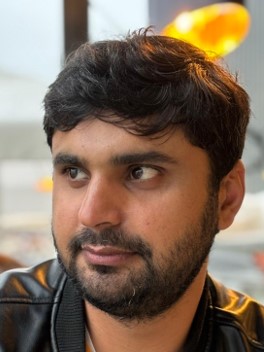
Dr. Khurram Tahir
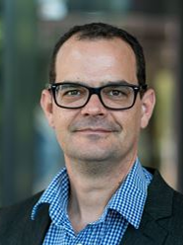
