P27 - Plant water uptake
Rhizosphere functions in plant water uptake: Mechanistic link between carbon and water fluxes in the plant-soil system
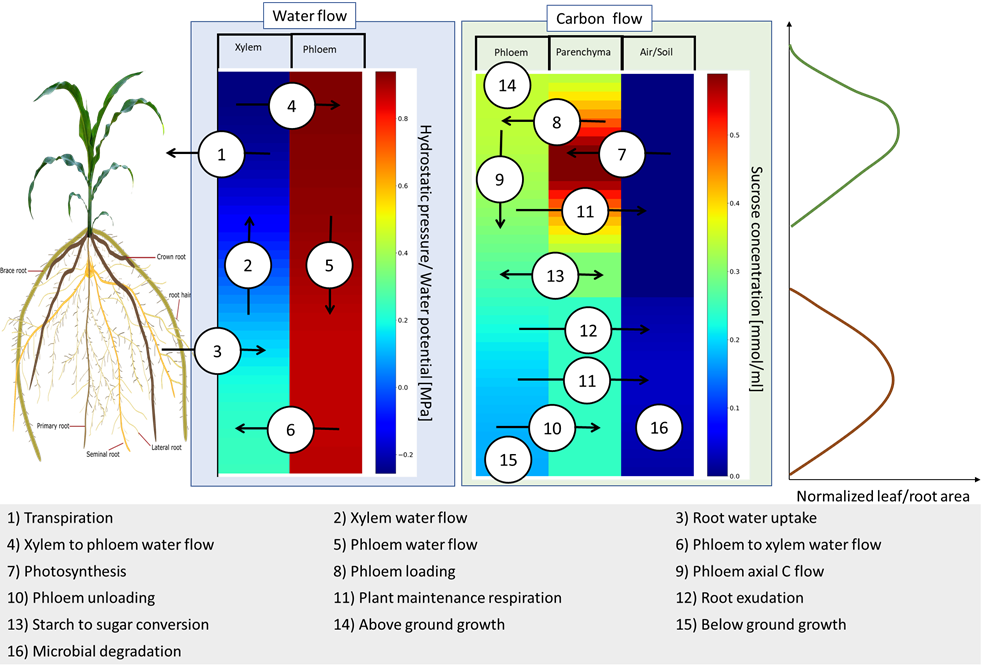
The root architecture of a plant is the result of the tradeoff between maximizing the soil explored for water and nutrients and minimizing the cost of carbon (C) and energy needed for root development and maintenance. Crops such as cereals develop complex root systems consisting of several root types. Given such complexity, it is fundamental to understand why plants develop such complex root systems. What is the contribution of different root types, root segments and rhizosphere properties to water and nutrient uptake, particularly under water deficit conditions? Individual roots have varying contributions to water uptake from the soil; thus, it is vital to understand whether plants promote elongation and production of a particular root type under varying soil conditions (e.g., soil moistures and textures).
The general objective of this project is to link the pattern of C allocation into different root segments (i.e., root type, root age and locations) and their rhizosphere to the functioning of roots in water acquisition from the soil. We will consider different water contents to assess the effect of soil drying, as the major soil physical constraints to plant production, on the spatiotemporal pattern of C allocation across the soil-plant-atmosphere continuum, and root water uptake. The main hypothesis is, that under varying soil conditions (moisture and texture), plants allocate more C to the production and maintenance of individual roots (type and regions) that have greater functionality in water uptake.
We plan to quantify root growth and water flux along root-shoot systems of soil-grown plants using a neutron radiography technique combined with injection of D2O. These results will be combined with modeling of water flow and microscopic cross-sections of roots and shoots to estimate the profile of hydraulic conductivities. In parallel, we will mechanistically quantify C fluxes across the soil-plant-atmosphere continuum using a combination of 13C and 14C labeling experiments.
Outcome
Project 27 of the SPP2089 entered its second phase with a focus on linking the pattern of carbon (C) allocation across different root segments (including root type, age, and location) and their associated rhizosphere to root functioning, particularly in relation to water acquisition from soil. This comprehensive approach aimed to better understand how roots adapt to various environmental conditions to optimize water uptake.
The first study investigated the root water uptake of maize wild-type plants grown in contrasting soil conditions, specifically varying soil texture and moisture levels. Neutron radiography, in combination with deuterated water (2H2O) labeling, was employed to quantify root water uptake in situ. The collected data is currently undergoing analysis and processing.
The second study focused on the development of the rhizosphere’s hydraulic properties in maize plants grown under different soil conditions. Neutron radiographs were taken over three consecutive days, and the soil moisture around newly emerged roots was measured. This research highlights the dynamic nature of maize plants in adjusting the hydraulic properties of their rhizosphere. By responding to external factors, particularly soil texture and moisture, the plants aim to optimize root-soil contact. As a result, a more pronounced rhizosphere is found in coarser soil textures, showing interactions with the soil moisture (
Adamczewski et al., 2024
).
The third study aimed to investigate how recently assimilated carbon (C) is partitioned across different root types, segments, and the rhizosphere of maize plants under varying soil conditions. Using 13C pulse labeling, we quantified the carbon fluxes across the soil-plant-atmosphere continuum. Additionally, 14C labeling, coupled with phosphor imaging, was employed to visualize the distribution of newly assimilated carbon to both the root system and the rhizosphere. The findings revealed that soil conditions influence carbon partitioning. Specifically, maize plants in dry soils allocated more carbon to aboveground biomass. Soil texture also played a role in carbon distribution, with coarser soils leading to a greater allocation of carbon to the root system. Across all treatments, crown roots consistently received the most carbon, followed by seminal and lateral roots. Notably, in the dry treatments, a relatively higher proportion of carbon was directed to lateral roots.
In the fourth working package, we plan to integrate these findings and model carbon partitioning across the soil-plant-atmosphere continuum. This will be coupled with a root water uptake model to further explore the interrelationships between water and carbon dynamics in maize plants.
Link to English scientific abstract
Link to German scientific abstract